Abstract
Background: Tribulus terrestris L. is a traditional herb regularly recognised as puncture vine, yellow vine, devils horn, goat head and caltrop, this is often a yearly shaggy herbaceous plant species with stems of up to 2m long, having a place to the family of Zygophyllaceae.
Aim: The study explored the therapeutic potential of this herb as it is being utilised for pharmaceutical purposes because of its furostanol saponins, which have a stimulating impact on characteristic testosterone levels.
Setting: The study took place in different locations of Mpumalanga Province, Bushbuckridge (24.8398°S, 31.0464°E), Kamagugu (25.4566° S, 31.0034° E) and Nkomatipoort (25.4510° S, 31.9587° E).
Methods: Utilising ultra-high-performance liquid chromatography quadrupole time-of-flight mass spectrometry (UPLC-QTOF/MS), 50 metabolites were tentatively identified within the leaves of Tribulus terrestris L. from three diverse areas of Mpumalanga Province, South Africa.
Results: Metabolomic-chemometric analysis revealed that Parvispinoside B, F-Gitonin and Gitonin scored highest for the discrimination of Tribulus terrestris L. from three locale clusters. Heat maps showed designs and groupings based on the metabolite concentrations.
Conclusion: This study provided novel insights in terms of thorough identification of the secondary metabolites and characterization of the leaves of Tribulus terrestris L. in the areas studied.
Contribution: To the best of our knowledge, this study presents the first metabolite profile of Tribulus terrestris L. and its compositional differences in the Mpumalanga region, providing chemical-based evidence for its nutritive and/or health benefits
Keywords: gitonin; steroidal saponins; chemometric; UPLC-QTOF/MS; medicinal plants; PCA; heat map.
Introduction
Tribulus terrestris L. is a traditional herb regularly recognised as puncture vine, yellow vine, devil’s horn, goat head and caltrop; this is often a yearly shaggy herbaceous plant species with stems of up to 2 m long. It belongs to the family of Zygophyllaceae (Hashim et al. 2014; Zhu et al. 2017). T. terrestris L. is an indigenous plant species that thrive in warm, humid climates in Africa, southern Europe, Australia and western and southern Asia (Hashim et al. 2014). In addition, it is broadly spread throughout Africa, Japan, Korea, some parts of Asia and China (Sharifi, Darabi & Akbarloo 2003). T. terrestris L. grows best in light-textured soils and is found in abandoned areas and along the roadside, cultivated crops, gardens, and overgrazed pasture (Hashim et al. 2014). The plant is well-known for its yellow petal bloom and prickly natural product, while the leaves are pinnate, short and inverted, with 4–8 sets of spear-shaped leaflets on each leaflet. The fruits have a rough surface, are axe-shaped and are normally 6 mm long, with lengths ranging from 7 mm to 12 mm (Zhu et al. 2017).
The seeds are encased in carpels (a woody star-shaped structure) around 5 mm – 7 mm and 5 mm – 6 mm in length and width, respectively. Each carpel comprises approximately five seeds, and each seed is yellow and almost 3 mm long. The root is stringy, slim, round, hollow, frequently branched, light brown and bearing numerous small rootlets (Chhatre et al. 2014). T. terrestris L. is used as a palliative, tonic, stomachic, antihypertensive, sexual enhancer, urinary disinfectant, astringent, diuretic and lithotriptic in traditional medicine (Chhatre et al. 2014). The fruit of T. terrestris L. has been employed in Chinese pharmacopoeia as a cough expectorant that improves eyesight, as a diuretic and for tonifying the kidney, as a cure for skin itch, vertigo and headache, and as a treatment for mammary duct obstruction (Zhu et al. 2017). In India, however, it is used to treat infertility, impotence, low libido, and erectile dysfunction.
Numerous pharmacological studies have revealed T. terrestris L. to have antimicrobial activity, antiurolithic activity, diuretic activity, aphrodisiac activity, antidiabetic activity, immunomodulatory activity, antioxidant activity absorption enhancement and central nervous system activity (Chhatre et al. 2014; Hashim et al. 2014; Kianbakht & Jahaniani 2003). Moreover, it was detailed that the antimicrobial activity of T. terrestris L. varies with the plant part utilised and its origin. For instance, the spirostanol saponins of Iranian and Indian T. terrestris L. were reported to exhibit antifungal activity against Cryptococcus neoformans and Candida albicans. In addition, the leaves, fruit and roots of Iraqi T. terrestris L. were stated to have antimicrobial activity against 11 species of pathogenic and nonpathogenic micro-organisms’ rootlets (Chhatre et al. 2014). The dominant saponins of T. terrestris L. are thought to be spirostanol and furostanol. T. terrestris L.’s steroidal saponins are thought to be the plant’s active ingredients, and the amount and variety of these compounds mostly depend on the environment (Dinchev et al. 2008). The saponins of T. terrestris L. from different geographic areas vary in terms of composition and content (Kostova & Dinchev 2005). To date, 58 and 50 kinds of spirostane and furostane saponins have been recognised from T. terrestris L., respectively, while flavonoids in T. terrestris L. are the derivatives of quercetin, isorhamnetin and kaempferol (Yang et al. 2014). For example, kaempferol-3-O-rutinoside, quercetin-3-O-gent-7-O-glu and isorhamnetin-3-O-gent-7-O-glu are flavonoids with kaempferol, quercetin and isorhamnetin as the basic parent structure, respectively, while tribulusimide C, harmine, tribulusterine, tribulusin A, etc. are the main alkaloids isolated from the leaves, fruit and stems of T. terrestris L. (Ren et al. 1994). Among the distinctive sort of compounds, flavonoids and steroidal saponins are observed as the foremost crucial metabolites with different bioactivities. Chemometric analysis is widely used in metabolomics research to characterise and assess the diversity of bioactive metabolites found in useful foods. Furthermore, a phytochemical database for bioactive compounds in foods that can be linked to chemical properties associated with nutritional and nutraceutical effects is required (Farag et al. 2019). As a result, the purpose of this study was to look into the various metabolites in T. terrestris L. from different locations in Mpumalanga province using ultra-high-performance liquid chromatography (UHPLC) coupled with a high-resolution quadrupole time-of-flight mass spectrometry (Q-TOF-MS) technique and a chemometric analysis approach.
Research methods and design
Plant material
Fresh leaf samples of T. terrestris L. were separately harvested from three different locations of Mpumalanga province, Bushbuckridge (24º50’23.28’’S, 31º2’47.04’’E), Kamagugu (25º27’23.76’’S, 31º0’12.24’’E) and Nkomatipoort1 (25º27’3.6’’S, 31º57’31.32’’E). The samples were harvested between October and December 2020. All the harvested samples were growing in the locations as weeds (not cultivated). To minimise variation, young tender leaves, specifically from the apex of the shoot, free of dirt or soil particles, decay and/or damage, were carefully plucked from 20 young flowering plants according to the method reported by Ntuli (2019), with modifications. To prevent contamination and moisture loss, the samples were put in Ziploc™ bags. On arrival in the laboratory, the samples were separately washed under running distilled water and freeze-dried (−85°C, LyoQuest-55/Telstar, Shanghai, China) and ground into fine powder for analysis according to the method reported by Moloto et al. (2020).
Extraction of leaf extracts for ultra-high-performance liquid chromatography quadrupole time-of-flight mass spectrometry analysis
Freeze-dried leaf samples (0.1 g) were homogenised in water with 2 mL of 80% methanol, then sonicated at 30°C for 30 min. The mixture was then centrifuged at 3000 × g for 20 min at 4°C using a Hermle Z326k (Hermle Labortechnik GmbH, Wehingen, Germany). The collected supernatants were used for the UHPLC-QTOF-MS analysis.
The ultra-high-performance liquid chromatography quadrupole time-of-flight mass spectrometry profiling and quantification
A 0.22-µm polytetrafluorethylene filter was then used to filter the supernatants. A Quadrupole 120 time-of-flight (QTOF) mass spectrometer UPLC-QTOF/MS (Waters, Milford, Massachusetts, United States of America [USA]) was used to identify and quantify predominant secondary metabolites. An ACQUITY UPLC BEH C18 column (2.1 mm × 100 mm i.d., 1.7 × 10−6 m; Waters) was used for all analyses. The mobile phase was composed of acetonitrile (A) and 0.1% formic acid, v/v (B), with the following gradient elution: (1) 0–8 min, 95% – 80% A; (2) 8–12 min, 80% – 70% A; (3) 12–15 min, 70 A – 65 A; (4) 15–18 min, 65% A; (5) 18–21 min, 65% – 20% A; (6) 21–23 min, 20% – 5% A; (7) 23–24 min, 5% A; (8) 25–30 min, 95% A. The flow rate of the mobile phase was 0.4 mL/min and the temperatures of the column and autosampler were maintained at 30°C and 10°C, respectively. Data were processed using Mass Spectrometry – Data Independent Analysis (MS-DIAL) and MS-FINDER (RIKEN Center for Sustainable Resource Science: Metabolome Informatics Research Team, Kanagawa, Japan) (Lai et al. 2018; Tsugawa et al. 2015). Functions 1 (unfragmented channel) and 2 (fragmented channel) of the Waters MSe data were processed by MS-DIAL to produce MS1 and MS2 spectra as well as extracted ion chromatograms with associated peak height intensity data. As calibration standards are not available for the majority of these compounds, the peak height intensity was converted to concentration in a semiquantitative manner by interpolation of a calibration curve for catechin acquired under the same instrumental conditions. Each deconvoluted feature (alignment in MS-DIAL), together with its associated MS1 and MS spectra, were exported from MS-DIAL to MS-FINDER. Based on the accurate mass elemental compositions, possible compounds were identified from the listed databases and then subjected to in silico fragmentation. According to the spectral match between the in silico and measured spectra, a score (out of 10) is assigned to each of the possible compound matches, with the highest score being accepted as the most likely (assuming a score of at least four).
Chemometric data analysis
The UPLC-QTOF-MS data of T. terrestris L. from different locations of Mpumalanga province were analysed with MetaboAnalyst 5.0 (Xia Lab @ McGill, Ste-Anne-de-Bellevue, Quebec, Canada) using principal component analysis (PCA), partial least squares – discriminant analysis (PLS-DA) and variable importance in projection (VIP) scores and a heat map. To evaluate the PLS-DA model’s goodness-of-fit, Q2 (predictive ability) and R2 (cumulative interpretation ability) were taken as initial indicators. Using a VIP plot, significantly different metabolites were screened.
Ethical considerations
This article followed all ethical standards for research without direct contact with human or animal subjects.
Results and discussion
Identification and characterisation of secondary metabolites using ultra-high-performance liquid chromatography quadrupole time-of-flight mass spectrometry
Secondary metabolites that could be the elements driving the antiproliferative effect of T. terrestris L. leaves were identified by nontargeted profiling using ultra-performance liquid chromatography coupled with high-resolution Q-TOF-MS. The UHPLC mass spectrometry (MS) examination of T. terrestris L. leaves taken from several locations in Mpumalanga province revealed 50 secondary metabolites, the majority of which were flavonoids, phenolic acids and saponins (Table 1). The annotations or identifications were tentative based on accurate mass elemental composition and fragmentation spectra. Based on ESI positive data, the results from this study revealed that quercetin derivatives (quercetin 3-gentiobioside and rutin) and F-gitonin with concentrations of 10 886, 9307 and 7296.7 mg/kg DW, peak 7; 9068, 7118 and 5885.3 mg/kg DW, peak 9; and 10 387, 7613.5 and 5707.7 mg/kg DW, peak 23 in Kamagugu, Nkomati and Bushbuckridge, respectively, were predominant compounds in the leaves of T. terrestris L. Peak 39, m/z 426.301, was discovered as compound jervine in the leaves of T. terrestris L., which had the lowest concentrations (296.7, 440, and 422.5 mg/kg DW) from Bushbuckridge, Kamagugu and Nkomati, respectively. The leaves of T. terrestris L., as reported in this study, were high in steroidal saponins, which are compounds likely to be responsible for the libido effects in humans and possibly the testosterone mimicry in animals (Ștefănescu et al. 2020). Enrichment of the saponins in T. terrestris L. reported in this study poses them as an ideal carbohydrate source for diabetic patients because of their ability to potentiate glucose-induced insulin release (Pereira-Leal & Seabra 2001). Saponins, because of their amphiphilic molecule, have membrane-permeabilising properties; thus, they could increase the absorption of other compounds. This property is of great importance because toxic effects could appear in patients with multiple conditions who undergo chronic treatments. The current findings contrast with those of Lazarova et al. (2011), who found significant differences (different amounts of chemicals and the absence of some compounds) between samples taken from the same geographic location. A rise in the use of T. terrestris L. supplements has also been seen among athletes, who are always looking for natural ways to improve their performance (Ștefănescu et al. 2020). Saponins have biological and therapeutic activities that include: (1) haemolytic factor, (2) anti-inflammatory, (3) antibacterial, (4) antifungal, (5) antiviral, (6) insecticidal, (7) anticancer, (8) cytotoxic and (9) molluscicidal effects (Abdel Gawad 1999; Armelle et al. 2018; Cheng et al. 2011; Ellen et al. 2007; Hassan et al. 2010; Just et al. 1998; Simões, Amoros & Girre 1999; Sindambiwe et al. 1998; Sparg, Light & Van Staden 2004).
TABLE 1: Tentative identification of secondary metabolites in Tribulus terrestris from three different locations of Mpumalanga province using ultra-high-performance liquid chromatography quadrupole time-of-flight mass spectrometry data. |
As a result of the complexity and multidimensionality of metabolomic data, appropriate statistical and chemometric tools were used to obtain chemical information and convert it into biological knowledge (Boccard & Rudaz 2014). Chemometrics is the science of extracting valuable information from complex data sets through pattern recognition and machine learning algorithms (Brereton 2015; Tebani, Afonso & Bekri 2018). The unsupervised (PCA) obtained data from the UPLC-QTOF/MS showed that the phenolic metabolites in the leaves of T. terrestris L. from different locations of Mpumalanga province differed in distribution. Principal component analysis is a multivariate technique that increases interpretability and minimises the loss of biological information by reducing the dimensionality of complex data sets (Jolliffe & Cadima 2016). The underlying structures and characteristics of the data are thus revealed by this unsupervised, explorative method. The PCA plot from this study revealed three district clusters based on the metabolites shown by the leaves of T. terrestris L. in different locations of Mpumalanga province (Bushbuckridge, Kamagugu and Nkomati village) (Figure 1 and Figure 2). In this study, the sample size was too small to develop robust chemometric models. However, as the aim was not to develop a prediction model but merely to investigate inter- and intraspecies variation, chemometric analysis was nevertheless used to analyse the liquid chromatography mass spectrometry (LC-MS) data. The two principal component (PC) models were able to distinguish clearly between the locations, with 22.9% separation along PC2 and 37.6% separation along PC1. Two locations, namely Bushbuckridge and Kamagugu, were distinctly separated by the first principal component (PC1), with Bushbuckridge samples situated on the negative (PC1) and Kamagugu on the positive (PC1) areas of the plot, while one sample from the Nkomati locality clustered along the negative (PC1), and the rest clustered in the positive (PC1). The relationship of the observed variation to locality was further evaluated by performing classification and discriminant analysis using orthogonal projections to latent structures discriminant analysis (OPLS-DA). This supervised pattern recognition technique was used to identify variables (in this case, peaks on the chromatogram) responsible for the differences between the groups. To obtain more in-depth information regarding the differential metabolic profiles revealed by PCA modelling, a supervised method, OPLS-DA, was used. It can be seen from the plot that the greatest variation was along PC2 (Figure 3). The OPLS-DA scores plot confirmed that the samples from different locations were very different in composition. Figure 4 shows the box-and-whisker plot representing an in-depth, semiquantitative visualisation of the distribution of selected metabolites ([A] parvispinoside B, [B] F-gitonin and [C] gitonin) in the leaves of T. terrestris L. from three different locations of Mpumalanga province plots. The interlocation variations in metabolite profiles are illustrated by the increased or relative decrease of the mentioned metabolites. Figure 4 reveals a relative increase in the metabolite concentration of T. terrestris L. leaves from the Bushbuckridge region, while the leaves from the Kamagugu region revealed a decreased concentration of these metabolites. The location-based quantitative and qualitative metabolite distribution was further evidenced in the group-wise metabolite selection, biplot-based discrimination analysis and the variable importance in projection (VIP), showing metabolic markers contributing to metabolic phenotypes in Figure 4. These are secondary metabolites, which were responsible for the differentiation in PCA detected. The performance of the developed model was validated by the computed parameters ‘R2 (0.9856)’ and ‘Q2 (0.9577)’, indicating its covered variance and good prediction power, respectively (Figure 5). Metabolites facilitating the discrimination of the leaves of T. terrestris L. from three different locations are shown in Figure 4. The PLS-DA also finds the characteristics (metabolites) with the highest VIP scores. As a result, the contribution of each metabolite to group separation was calculated using VIP ratings. Variable importance in projection scores is calculated by adding the squares of the partial least squares (PLS) loadings, which measure the amount of Y-variance explained across all dimensions and the weighted sum of the PLS-regression coefficients (Carvalho et al. 2021). Thus, metabolites were ranked according to their VIP scores, and only the top metabolites with the highest VIP scores were deemed to provide the most meaningful interpretation of the data (Carvalho et al. 2021). Hierarchical cluster analysis was performed on the whole data set of discovered metabolites to visualise clusters of samples with comparative chemical composition, which gave additional evidence of the related metabolites associated with T. terrestris L. from different regions. The results of this study were merged with a heat map structure created from metabolite concentrations across all samples (Figure 6). Blue in the heat map denotes metabolite downregulation or lower expression, whereas red depicts metabolite upregulation or higher expression. Heat maps also make patterns that might not be evident or visible.
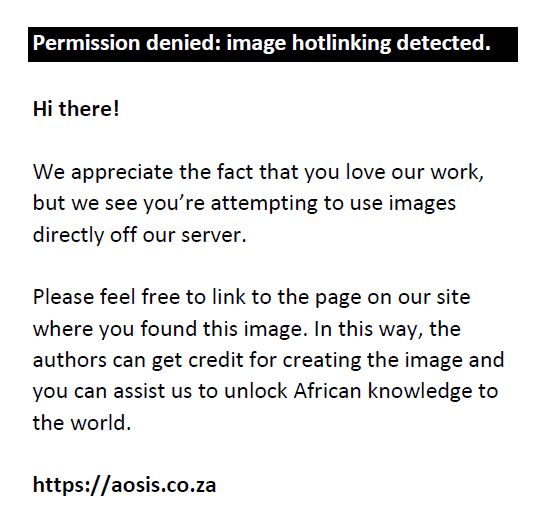 |
FIGURE 1: Box plot showing (a) parvispinoside B, (b) F-gitonin and (c) gitonin detected in the leaves of Tribulus terrestris from three different locations of Mpumalanga province. |
|
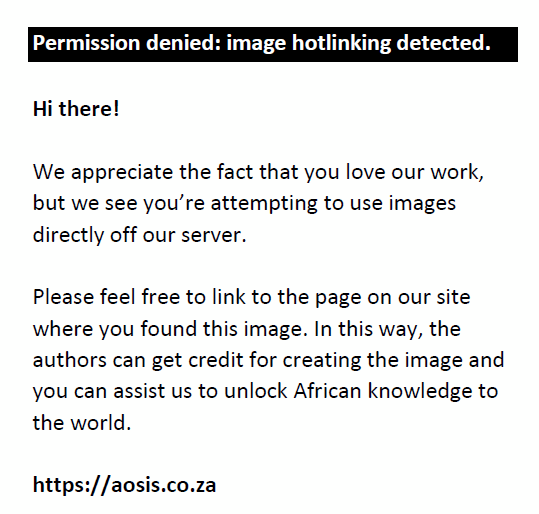 |
FIGURE 2: Principal component analysis score plot of principal component1 versus principal component2 scores of Tribulus terrestris from three different locations in Mpumalanga province, analysed via ultra-high-performance liquid chromatography quadrupole time-of-flight mass spectrometry. |
|
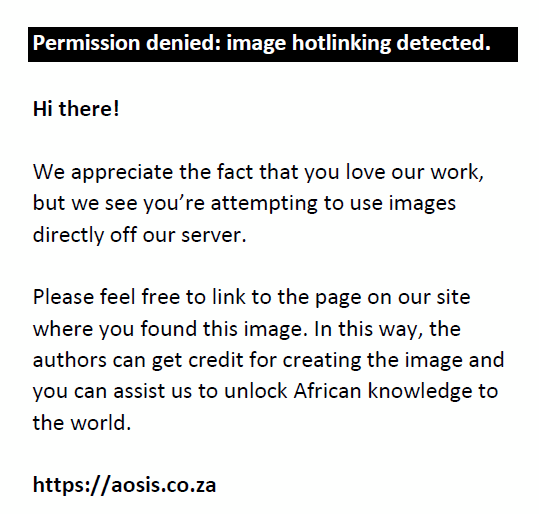 |
FIGURE 3: The score plot of orthogonal partial least squares discriminant analysis (OPLS-DA) of Tribulus terrestris from three different locations in Mpumalanga province. |
|
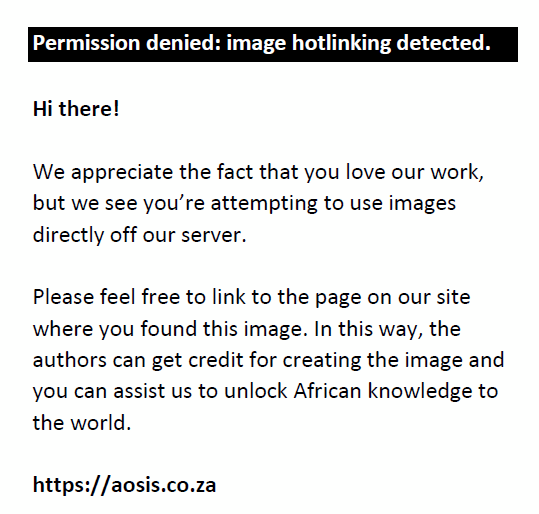 |
FIGURE 4: The identification results of chemical markers of Tribulus terrestris from three different locations of Mpumalanga province (VIP > 3). |
|
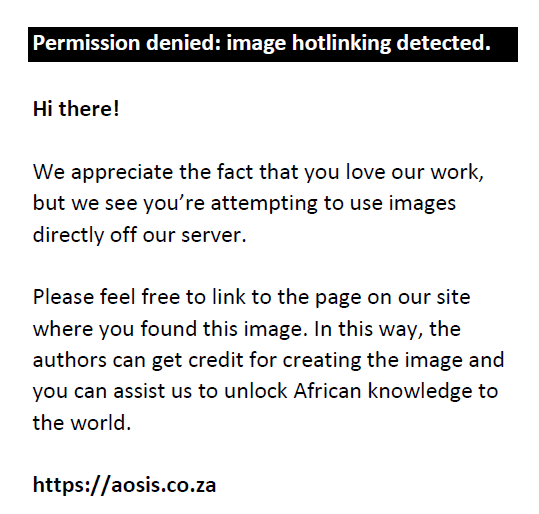 |
FIGURE 5: Cross validation to evaluate the PLS-DA model’s goodness-of-fit, Q2 (predictive ability) and R2 (cumulative interpretation ability) were taken as initial indicators T. terrestris from three different locations in Mpumalanga province. |
|
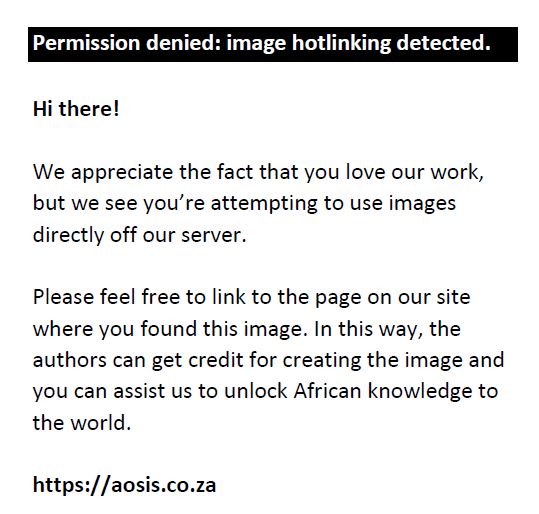 |
FIGURE 6: Heat map of 25 phenolic metabolites quantified in leaves of Tribulus terrestris from three different locations of Mpumalanga province organised in hierarchical clustering. |
|
Conclusion
The results of this study indicate that the leaves of T. terrestris are a rich source of secondary metabolites, justifying its use in folk medicine to cure a range of diseases in various countries. The significant number of steroidal saponins in this plant is a distinctive feature. T. terrestris from different geographical regions had diverse secondary metabolite compositions and concentrations. According to the findings of this study, the leaves of T. terrestris contain therapeutic compounds and could be used to treat ailments. However, more research is needed to fully understand the pharmacological properties and biological activities associated with the described metabolite molecules.
Acknowledgements
The authors wish to acknowledge the National Research Foundation (NRF) for financial assistance in conducting this research. The authors would also like to thank the School of Biology and Environmental Science, University of Mpumalanga, for their immense contributions towards achieving this research work.
Competing interests
The authors declare that they have no financial or personal relationships that may have inappropriately influenced them in writing this article.
Authors’ contributions
The authors designed the study, coordinated data collection, carried out all the field work and drafted the manuscript.
Funding information
The NRF supported this study.
Data availability
Data sharing is not applicable to this article as no new data were created or analysed in this study.
Disclaimer
The views and opinions expressed in this article are those of the authors and do not necessarily reflect the official policy or position of any affiliated agency of the authors.
References
Abdel Gawad, M.M., El Sayed, M.M. & Abdel Hameed, E.S., 1999, ‘Molluscicidal steroidal saponins and lipid content of Agave decipiens’, Fitoterapia 70(4), 371–381. https://doi.org/10.1016/S0367-326X(99)00057-X
Boccard, J. & Rudaz, S., 2014, ‘Harnessing the complexity of metabolomic data with chemometrics’, Journal of Chemometrics 28(1), 1–9. https://doi.org/10.1002/cem.2567
Brereton, R.G., 2015, ‘Pattern recognition in chemometrics’, Chemometrics and Intelligent Laboratory Systems 149, 90–96. https://doi.org/10.1016/j.chemolab.2015.06.012
Carvalho, F.V., Santana, L.F., Da Silva, V.D.A., Costa, S.L., Zambotti-Villelae, L., Colepicolo, P. et al., 2021, ‘Combination of a multiplatform metabolite profiling approach and chemometrics as a powerful strategy to identify bioactive metabolites in Lepidium meyenii (Peruvian maca)’, Food Chemistry 364(5), 130453. https://doi.org/10.1016/j.foodchem.2021.130453
Cheng, T.C., Lu, J.F., Wang, J.S., Lin, L.J., Kuo, H.I. & Chen, B.H., 2011, ‘Antiproliferation effect and apoptosis mechanism of prostate cancer cell PC-3 by flavonoids and saponins prepared from Gynostemma pentaphyllum’, Journal of Agricultural and Food Chemistry 59(20), 11319–11329. https://doi.org/10.1021/jf2018758
Chhatre, S., Nesari, T., Somani, G., Kanchan, D. & Sathaye, S., 2014, ‘Phytopharmacological overview of Tribulus terrestris’, Pharmacognosy Reviews 8(15), 45. https://doi.org/10.4103/0973-7847.125530
Dinchev, D., Janda, B., Evstatieva, L., Oleszek, W., Aslani, M.R. & Kostova, I., 2008, ‘Distribution of steroidal saponins in Tribulus terrestris from different geographical regions’, Phytochemistry 69(1), 176–186. https://doi.org/10.1016/j.phytochem.2007.07.003
Ellen, D., Ellen, L., Danny, G. & Guy, S., 2007, ‘Novel advances with plant saponins as natural insecticides to control pest insects’, Pest Technology 1(2), 96–105.
Farag, M.A., Khattab, A.R., Maamoun, A.A. & Heiss, A.G., 2019, ‘UPLC-MS metabolome based classification of Lupinus and Lens seeds: A prospect for phyto-equivalency of its different accessions’, Food Research International 115, 379–392. https://doi.org/10.1016/j.foodres.2018.11.003
Hashim, S., Bakht, T., Marwat, K.B. & Jan, A.A., 2014, ‘Medicinal properties, phytochemistry and pharmacology of Tribulus terrestris L. (zygophyllaceae)’, Pakistan Journal of Botany 46(1), 399–404.
Hassan, S.M., Haq, A.U., Byrd, J.A., Berhow, M.A., Cartwright, A.L. & Bailey, C.A., 2010, ‘Haemolytic and antimicrobial activities of saponin-rich extracts from guar meal’, Food Chemistry 119(2), 600–605. https://doi.org/10.1016/j.foodchem.2009.06.066
Jolliffe, I.T. & Cadima, J., 2016, ‘Principal component analysis: A review and recent developments’, Philosophical Transactions of the Royal Society A: Mathematical, Physical and Engineering Sciences 374(2065), 20150202. https://doi.org/10.1098/rsta.2015.0202
Just, M.J., Recio, M.C., Giner, R.M., Cuéllar, M.J., Máñez, S., Bilia, A.R. et al., 1998, ‘Anti-inflammatory activity of unusual lupane saponins from Bupleurum fruticescens’, Planta Medica 64(5), 404–407. https://doi.org/10.1055/s-2006-957469
Kianbakht, S. & Jahaniani, F., 2003, ‘Evaluation of antibacterial activity of Tribulus terrestris L., growing in Iran’, Iranian Journal of Pharmacology and Therapeutics 2, 22–24.
Kostova, I. & Dinchev, D., 2005, ‘Saponins in Tribulus terrestris-chemistry and bioactivity’, Phytochemistry Review 4(2), 111–137. https://doi.org/10.1007/s11101-005-2833-x
Lai, Z., Tsugawa, H., Wohlgemuth, G., Mehta, S., Mueller, M., Zheng, Y. et al., 2018, ‘Identifying metabolites by integrating metabolome databases with mass spectrometry cheminformatics’, Nature Methods 15(1), 53–56. https://doi.org/10.1038/nmeth.4512
Lazarova, I., Ivanova, A., Mechkarova, P., Peev, D. & Valyovska, N., 2011, ‘Intraspecific variability of biologically active compounds of different populations of Tribulus terrestris L. (Zygophyllaceae) in South Bulgaria’, Biotechnology & Biotechnological Equipment 25(2), 2352–2356. https://doi.org/10.5504/BBEQ.2011.0040
Mbaveng, A.T., Ndontsa, B.L., Kuete, V., Nguekeu, Y.M., Çelik, I., Mbouangouere, R. et al., 2018, ‘A naturally occurring triterpene saponin ardisiacrispin B displayed cytotoxic effects in multi-factorial drug resistant cancer cells viaferroptotic and apoptotic cell death’, Phytomedicine 43(1), 78–85. https://doi.org/10.1016/j.phymed.2018.03.035
Moloto, M.R., Phan, A.D.T., Shai, J.L., Sultanbawa, Y. & Sivakumar, D., 2020, ‘Comparison of phenolic compounds, carotenoids, amino acid composition, in vitro antioxidant and anti-diabetic activities in the leaves of seven cowpea (Vigna unguiculata) cultivars’, Foods 9(9), 1285. https://doi.org/10.3390/foods9091285
Ntuli, N.R., 2019, ‘Nutrient content of scarcely known wild leafy vegetables from northern KwaZulu-Natal, South Africa’, South African Journal of Botany 127, 19–24. https://doi.org/10.1016/j.sajb.2019.08.033
Pereira-Leal, J.B. & Seabra, M.C., 2001, ‘Evolution of the Rab family of small GTP-binding proteins’, Journal of Molecular Biology 313(4), 889–901. https://doi.org/10.1006/jmbi.2001.5072
Ren, Y.J., Chen, H.S., Yang, J.Y. & Zhu, H., 1994, ‘Isolation and identification of a new derivative of cinnamic amide from Tribulus Terrestris’, Acta Pharmaceurica Sinica 29(3), 204–206.
Sharifi, A.M., Darabi, R. & Akbarloo, N., 2003, ‘Study of antihypertensive mechanism of Tribulus terrestris in 2K1C hypertensive rats: Role of tissue ACE activity’, Life Sciences 73(23), 2963–2971. https://doi.org/10.1016/j.lfs.2003.04.002
Simões, C.M.O., Amoros, M. & Girre, L., 1999, ‘Mechanism of antiviral activity of triterpenoid saponins’, Phytotherapy Research 13(4), 323–328. https://doi.org/10.1002/(SICI)1099-1573(199906)13:4%3C323::AID-PTR448%3E3.0.CO;2-C
Sindambiwe, J.B., Calomme, M., Geerts, S., Pieters, L., Vlietinck, A.J. & Vanden Berghe, D.A., 1998, ‘Evaluation of biological activities of triterpenoid saponins from Maesa lanceolata’, Journal of Natural Products 61(5), 585–590. https://doi.org/10.1021/np9705165
Sparg, S.G., Light, M.E. & Van Staden, J., 2004, ‘Biological activities and distribution of plant saponins’, Journal of Ethnopharmacology 94(2–3), 219–243. https://doi.org/10.1016/j.jep.2004.05.016
Ștefănescu, R., Tero-Vescan, A., Negroiu, A., Aurică, E. & Vari, C.E., 2020, ‘A comprehensive review of the phytochemical, pharmacological, and toxicological properties of Tribulus Terrestris L.’, Biomolecules 10(5), 752. https://doi.org/10.3390/biom10050752
Tebani, A., Afonso, C. & Bekri, S., 2018, ‘Advances in metabolome information retrieval: Turning chemistry into biology. Part II: Biological information recovery’, Journal of Inherited Metabolic Disease 41(3), 393–406. https://doi.org/10.1007/s10545-017-0080-0
Tsugawa, H., Cajka, T., Kind, T., Ma, Y., Higgins, B., Ikeda, K. et al., 2015, ‘MS-91 DIAL: Data-independent MS/MS deconvolution for comprehensive metabolome analysis’, Nature Methods 12(6), 523–526. https://doi.org/10.1038/nmeth.3393
Yang, F.K., Zhang, M.L., Quan, X.L., Xue, M.L., Cai, H.X. & Yang, J., 2014, ‘Determination and comparison HPLC of total flavonoids in different parts of Tribulus Terrestris glycoside content’, Modern Traditional Chinese Medicine 34(4), 61–64.
Zhu, W., Du, Y., Meng, H., Dong, Y. & Li, L., 2017, ‘A review of traditional pharmacological uses, phytochemistry, and pharmacological activities of Tribulus terrestris’, Chemistry Central Journal 11(60), 1–16. https://doi.org/10.1186/s13065-017-0289-x
Footnote
1. Nkomati, is short for Nkomatipoort and will be used throughout the article.
|