Abstract
Background: Lonchocarpus capassa is a medicinal plant used to treat diseases such as fungal infections, diarrhoea, oral candidiasis, and stomach complaints in South Africa.
Aim: The study aimed to investigate the cytotoxicity and antifungal compounds isolated from L. capassa leaf extracts.
Setting: The study was conducted in Muduluni village, Makhado Local Municipality, Limpopo province.
Methods: Leaf extracts were screened for antifungal activity against fungal pathogens: Candida albicans, Cryptococcus neoformans, and Aspergillus fumigatus. Bioassay-guided fractionation using column chromatography of the acetone extract led to the isolation of six antifungal compounds. Nuclear Magnetic Resonance spectroscopy and Mass Spectrometry were used for the identification of compounds. The antioxidant activity of the plant extracts was investigated using 1,1-diphenyl-2-picrylhydrazyl (DPPH) assays. Cytotoxicity of isolated compounds was determined using the 3-(4,5-dimethylthiazol)-2,5-diphenyl tetrazolium bromide (MTT) assay against Vero monkey kidney cells.
Results: The plant extract had an excellent minimum inhibitory concentrations (MIC) value of 40 μg/mL against the microorganisms. Compound 1 was identified as Lupeol, Compound 3 as Friedelin, and Compound 4 as 6-(γ,γ-Dimethylallyl)-3’,4’-dimethoxy-6”,6”-dimethylpyrano-[2”,3”:7,8]-flavanone (Compound 4). Compounds 2 and 5 were not identified because of the presence of mixtures of long-chain fatty acids. Friedelin was the most active radical scavenger in the DPPH assay. The compounds were not toxic with an LC50 value of ˃ 0.2 mg/mL.
Conclusion: Screening of medicinal plants could provide lead to the discovery of novel antifungal agents.
Contribution: The results support the traditional use of L. capassa to combat fungal infections in humans.
Keywords: Lonchocarpus capassa; antifungal activity; cytotoxicity; minimum inhibitory concentration; fungal infections.
Introduction
Fungal infections are the leading cause of morbidity and mortality all over the world, particularly in developing countries (Aumeeruddy-Elalfi, Gurib-Fakim & Mahomoodally 2016). The increase in resistance of fungal pathogens to the currently available antifungal drugs is a major health problem for public health care. Treatment of fungal infections is complicated because of Candida, Aspergillus, and Cryptococcus species that are resistant to the available antifungal agents (Sanguinetti, Posteraro & Lass-Florl 2015). The infection occurs because of the inhalation of blastospores and basidiospores which create a pulmonary infection in humans (Samie et al. 2019). Aspergillus fumigatus causes aspergillosis in humans through inhalation of airborne asexual spores called conidia (Kim 2016). Candida albicans causes candidiasis in humans, and it is found in the oral cavity, the gastrointestinal tract, and the female genital tract (Schmiedel & Zimmerli 2016). Currently, there are limited drugs such as polyene, itraconazole, and fluconazole available to treat fungal infections. However, some of the antifungal drugs are expensive, toxic, and have side effects (Zhang et al. 2020). Furthermore, it is difficult to find new antifungal agents because of resistant strain development among the fungal species against the existing antifungal drugs (Vallabhaneni et al. 2016). Therefore, new antifungal drugs that are cheaper, effective, non-toxic, and that are rapidly fungicidal are required to overcome these problems.
Medicinal plants used to treat fungal infections are assumed to be safe because of their long usage in traditional medicine. In particular, the bioactive compounds found in plants could be used as a source of new antifungal agents. These compounds form the basis for drug discovery that may combat various diseases in humans and are commercialised across the world. The use of natural products in drug discovery is a lead for finding new novel antifungal agents that may produce effective and curative agents for opportunistic fungal infections (Zhang et al. 2017). In a recent study, L. capassa was used in traditional medicine to treat fungal infections and related ailments in humans. In the preliminary screening, L. capassa had excellent antimicrobial activity with minimum inhibitory concentrations (MIC) of < 100 μg/mL, and antifungal compounds were present in the plant extracts.
Lonchocarpus capassa is a tree that grows up to 15 m tall belonging to the family Fabaceae. It is commonly known as ‘Mufhanda’ in Tshivenda. The tree is drought-tolerant and is distributed over Eastern Cape, KwaZulu-Natal, Mpumalanga, and Limpopo. The bark is grey, and the leaves are large and rough in texture (Van Wyk & Van Wyk 1997). The bark infusion is used to treat diarrhoea, colds, snake bites, intestinal problems, and hookworm, and the burnt root smoke is inhaled to treat colds (Kilonzo, Ndakidemi & Chacha 2016). The fine powder of stem bark of L. capassa is mixed with porridge for the treatment of wounds (Moshi, Kagashe & Mbwambo 2006). The root bark extracts are used to treat stomach aches and hookworms (Kokwaro 1993). The plant is used to treat sexually transmitted infections. The leaves are used to combat respiratory problems, chest pains, fever, and sexually transmitted infections. The plant has been reported to have antifungal and antibacterial activities (Kilonzo, Chacha & Ndakidemi 2017). Previously, it was found that the genus Lonchocarpus has the ability to produce compounds such as alkaloids, triterpenoids derived from benzoic acids, and flavonoids. Based on the literature, no reports on the chemical isolation and characterisation of constituents of L. capassa have been reported. In this paper, we investigated the presence of antifungal compounds of leaf extracts of L. capassa against the fungal pathogens C. albicans, C. neoformans and A. fumigatus.
Research methods and design
Plant collection
The plant species were collected in March 2021 from their natural habitat in Muduluni village, Makhado Local Municipality, Limpopo province. The plants were stored in open mesh orange bags at room temperature of 25 °C to ensure efficient drying of the material. Literature and Larry Leach Herbarium at the University of Limpopo were used to identify the plant species. Voucher specimens (TC 01) were prepared and deposited at the Herbarium. Leaf materials were allowed to dry for 3–5 weeks and ground to a fine powder (Figure 1).
Finely-ground leaf material (500 g) of L. capassa was serially extracted with 1500 mL of solvents of increasing polarities: hexane (non-polar), ethyl acetate (polar) dichloromethane (polar), acetone (polar), and methanol (polar). The combination of the solvent and the powder was left on a Labotec Model 20.2 shaking apparatus for 3 h. This allows the solvents to extract all the chemical components in the ground plant material. The extract was filtered through Whatman no.1 filter paper using a Büchner funnel. The solvents were concentrated using a rotary evaporator at 40 °C. The concentrated solvents were transferred into pre-weighed beakers and placed under a stream of cold air in a fume hood for complete dryness (Machaba 2018).
Solvent-solvent fractionation
Solvent-solvent fractionation method described by Adaramola and Onigbinde (2017) was used to separate fractions. The acetone crude extracts of L. capassa were separated using various solvents such as chloroform, n-butanol, acetone, and water. The acetone crude extracts were re-dissolved in acetone and transferred into a separatory funnel. A 500 mL of chloroform was added into the separatory funnel and shaken, then allowed to settle. The two layers were formed, and the bottom of the separatory funnel was opened to collect the aqueous fraction. The chloroform fraction was collected. Then, an equal volume of butanol was added to the water fraction into the separatory funnel and the butanol fractions were collected. In each step, the fractions were tested for antifungal activity using a serial dilution assay. The bioautography assay was used to determine the number of active compounds present in the fractions after each stage of solvent–solvent fractionation.
Phytochemical analysis
Aluminium-backed Thin Layer Chromatography (TLC) plates were used to analyse the chemical constituents of each plant extract (Mahlo, McGaw & Eloff 2013). Ten microlitres of each sample were loaded on TLC plates. The TLC plates were developed in three different eluent solvent systems: Ethyl acetate: methanol: water (EMW), Chloroform: ethyl acetate: formic acid (CEF), and Benzene: ethanol: ammonia hydroxide (BEA) (Kotze & Eloff 2002). Chemical components were visualised under visible and ultraviolet (UV) light (254 and 360 nm, Camac Universal UV lamp TL-600). Vanillin-sulphuric acid spray reagent (Stahl 1969) was used for the detection of chemical compounds not visible under UV light.
Fungal strains and inoculum quantification
C. albicans, C. neoformans and A. fumigatus were obtained from the Department of Veterinary Tropical Diseases at the University of Pretoria. The haemocytometer cell-counting method described by Aberkane et al. (2002) was used for counting the number of cells for each fungal culture. The sabouraud dextrose (SD) agar slants were used to grow the fungus for 7 days at 35 °C to prepare the inoculum of each isolate. The sterile loop was used to rub the slant and transfer it to a sterile tube with fresh SD broth (50 mL) (Mahlo et al. 2013). After shaking the sterile tubes for 5 min, appropriate dilutions were made to determine the number of cells by microscopic enumeration using a haemocytometer (Neubauer chamber; Merck S.A). The final inoculum concentration was adjusted to approximately 1.0× 106 cells/mL.
Structure elucidation
Nuclear Magnetic Resonance (NMR) was used for the identification of isolated antifungal compounds. All samples were sent to the Department of Chemistry at the University of Limpopo for NMR analysis and structure elucidation.
Bioautography assay
Thin-layer chromatography plates were loaded with 10 μL of each plant extract. The prepared plates were developed using different mobile systems of varying polarity: CEF, BEA, and EMW. The chromatograms were dried at room temperature under a stream of air overnight to remove the remaining solvent. Fungal cultures were grown on SD agar for 3–5 days. Cultures were transferred into an SD broth from agar with sterile swabs. The plates were incubated at 37 °C in a clean chamber at the humidified chamber overnight and further sprayed with 2 mg/mL solution of p-iodonitrotetrazolium (INT) violet and incubated for 2 h – 6 h to allow fungal growth. White areas indicated where reduction of INT to the coloured formazan did not take place because of the presence of compounds that inhibited the growth of the fungi (Begue & Kline 1972).
Column chromatography
The L. capassa acetone active fractions (5 g) resulting from solvent–solvent fractionation were subjected to column chromatography using silica gel as a stationary phase and various eluent solvent systems. Silica gel (200 g) was mixed with 500 mL hexane to form a slurry and packed in a glass column (denoted as column i) to a height of 50 cm and a diameter of 3 cm. The fractions were re-dissolved in different solvent mixtures such as hexane: ethyl acetate (4:1), (3:2), (2:3), and (1:4), and 100% methanol was used to elute the column (Mahlo et al. 2013). The column fractions were collected in test tubes and analysed on TLC plates. The prepared TLC plates were sprayed with vanillin-sulphuric acid spray reagent. The fractions were further analysed using a bioautography assay against the pathogenic fungi. Fractions with similar pure spots on TLC chromatograms were grouped together and evaporated to dryness under reduced pressure 45 °C. The combined fractions were further analysed using TLC fingerprinting. Fraction 20–22 resulting from column I chromatography was subjected to column II chromatography using silica gel as a stationary phase and various eluent systems. A column of 30 cm in height and 2 cm in diameter was used to pack a column. The fraction was re-dissolved in different solvent mixtures such as hexane: ethyl acetate (4:1), (3:2), (2:3), (1:4) (3:1) (96:4), (95:3) (94:2), (92:1), and (80:20). The fractions were collected into test tubes and analysed for TLC fingerprinting. The prepared TLC plates were sprayed with vanillin-sulphuric acid spray reagent.
Determining antifungal activity
Micro-dilution assay
Micro-dilution assay with some modifications was used to determine the antifungal activity of plant extracts, fractions, and isolated compounds (Masoko, Picard & Eloff 2005). The samples (100 μL) were serially diluted 50% with water in 96-well microtiter plates, and 100 μL of fungal culture was added to each well. Amphotericin B was used as the positive control and 100% acetone as the negative control. As an indicator of growth, 40 μL of 0.2 mg/mL p-iodonitrotetrazolium violet (INT) dissolved in water was added to the microplate wells. The microplates were covered and incubated for 3–5 days at 35 °C at 100% relative humidity after sealing in a plastic bag to minimise fungal contamination in the laboratory. The MIC values were recorded as the lowest concentration of the extract that inhibited antifungal growth.
Cytotoxicity assay
The cytotoxicity of plant crude extracts and isolated compounds were determined using the 3-(4,5-dimethylthiazol) - 2,5-diphenyltetrazoliumbromide (MTT) assay against Vero monkey kidney cells (Mosmann 1983; McGaw, Van Der Merwe & Eloff 2007). The cells were obtained from the Department of Veterinary Tropical Diseases (University of Pretoria). Minimal Essential Medium (MEM, Whitehead Scientific) was used to maintain the cells, supplemented with 0.1% gentamycin (Virbac) and 5% foetal calf serum (Highveld Biological). Two hundred μl of the cell suspension was pipetted into each sterile 96-well microtiter plate. The plates were incubated at 37 °C in a 5% CO2 incubator for 48 h with a test compound or extract. After incubation of 4 h in 30 μL of 5 mg/mL MTT solution, the MTT formazan crystals were dissolved by adding 50 μL DMSO to each well. The amount of MTT reduction was measured immediately by detecting absorbance in a microplate reader (Biotek Synergy) at a wavelength of 570 nm. Doxorubicin chloride was used as a positive control. The LC50 values were calculated as the concentration of the test compound resulting in a 50% reduction of absorbance compared to untreated cells.
Data analysis
The collected data were captured into MS Excel 2020 software and analysed using descriptive and inferential statistics.
Ethical considerations
The study was approved by the University of Limpopo Research Committee (TREC/115/2020).
Results and discussion
Acetone, dichloromethane (DCM), and methanol leaf extracts of L. capassa had excellent activity against C. neoformans and C. albicans with MIC values of 0.02 and 0.31 mg/mL. The average MIC values of the fractions differ, with the ethyl acetate fraction displaying the lowest average of 0.62 mg/mL (Table 1). The aqueous extracts were active against the tested fungal pathogens. These findings support the use of water as a good extractant by traditional health practitioners.
TABLE 1: Minimum inhibitory concentration values of the selected plant species against A. fumigatus, C. neoformans and C. albicans. The results are the mean of three replicates and the standard deviation was zero. |
Compound 1
The 1H NMR spectrum showed the presence of seven methyl protons at δH 0.77, 0.81, 0.86, 0.92, 0.95, 1.05, and 1.66 (integrated for 3H-each). A sextet of one proton at δH 2.38 was assigned as 19H. The H-3 proton showed a doublet of doublets at δH 3.19, while a pair of broad singlets at δH 4.55 and δH 4.67 (1H, each) was indicative of olefinic protons at (H-29 a & b).
A search in the dictionary of natural products and chemical abstracts services (Scifinder) using the spectroscopic information led to the structure of 1 lupeol (Figure 2), which was confirmed by comparing with the literature data (Ain & Bari 2010; Fotie et al. 2006). The structural assignment of Compound 1 was further substantiated by the 13C NMR experiments which showed seven methyl groups at [δc: 27.39 (C-23), 18.02 (C-28), 16.15 (C-25), 15.97 (C-26), 15.40 (C-24), 14.20 (C-27) and 19.31 (C-30)]; the signals because of an exomethylene group at [δc: 109.36 (C-29) and 151.04 (C-20)]; 10 methylene, 5 methine, and 5 quaternary carbons were assigned with the aid of Distortionless Enhancement by Polarisation Transfer (DEPT) experiment. The de-shielded signal at δc 79.04 was because of C-3 with a hydroxyl group attached to it.
The confirmation of the structure of M-C1 was accomplished through the 2D NMR experiments (Correlation spectroscopy [COSY], Heteronuclear single quantum coherence [HSQC] and Heteronuclear multiple-bond correlation [HMBC]). The HSQC spectrum of Compound 1 showed a strong correlation between two olefinic protons (H29 a,b) at δH 4.55 and 4.70 with a carbon peak at δc 109.36 (C-29). The proton resonance at δH 3.19 (C-3) corresponded with a carbon peak at δC 79.04 (C-3) ppm in the HSQC. Through HSQC, a sextet of one proton at δ 2.38 assigned as 19H showed a good correlation with carbon δc 48.00 (C-19). The COSY spectrum of Compound 1 exhibited some correlation between δH 2.37, H-19 and methylene proton signal (δH 1.37, H-21), also another methine proton signal (δH 1.89, H-18). Correlation between the oxygenated methine proton signal (δH 3.2, H-3) and methylene signal (δH 1.60, H-2) was also observed through COSY.
In the HMBC spectrum, the methine proton signal at δH 3.2 (H-3) showed cross peaks with a methyl carbon signal (δc 27.39, C-23) and a methyl carbon signal (δc 18.32, C-6). The sextet methyl signal at δH 2.37 (H-19) showed cross peaks with a methyl carbon signal [δc 19.31 (C-30)] and a quaternary carbon signal [δc 151.04 (C-20)]. The pair of broad olefinic proton at δH 4.55 and 4.70 showed cross peaks with a methylene carbon signal [δc 48.00 (C-19) and δc 19.31 (C-30)].
Lupeol belongs to bioactive triterpenoids with great therapeutic agents (Saxena et al. 2023). The isolated chemical structure of Lupeol (Figure 2) is in good agreement with the structure isolated by Jain and Bari (2010) from Wightia tinctoria. Lupeol was previously isolated from Holarhena floribunda (Fotie et al. 2006) and Axinella infundibuliformis (Lutta et al. 2008). The compound Lupeol was currently isolated from Sideroxylon mascatense (Ali et al. 2022), Croton echioides (Vendruscolo et al. 2022). Lupeol has shown good antioxidant activity and antibacterial activity was also reported (Ali et al. 2022). The cytotoxicity of Lupeol isolated from C. echiodes was reported (Vendruscolo et al. 2022). The anti-inflammatory, anti-tumour, anti-prostate, and antiprotozoal activities of Lupeol were reported (Margareth & Miranda 2009; Saxena et al. 2017; Wal et al. 2011).
Compound 3
The ¹H, 13C NMR had a signal for Ha-1 at δH 1.61 and Hb-2 at δ 2.27 integrating for 1 proton each. A signal for H-4 was observed integrating for one proton at δH 2.33, while H-6 was at δ 1.24 with corresponding C-2 and C-6 at δC 33.65 and 31.95, respectively. Eight signals typical of methyl protons were also observed at δ 0.80, 0.84, 0.92, 0.95, 1.00,1.02 and 1.03, 1.23 with corresponding 13C NMR signals at δC 29.73, 29.47 for Me-29 and Me-30, and 22.65 for Me-26. Signals typical of methylene carbons were also observed at δ 56.00, 55.50, and 59.52 for C-4, C-8, and C-10, while quaternary carbon signals were observed at δ 29.27 and 29.39 for C-20 and C-17. Also, typical signals of methylene carbons were observed at δ 14.16, 22.65, 28.00, 29.09, 31.95, 33.65, 35.64, 35.92, 36.33, 39.51. The cross correlation between a methyl signal at δ 1.24 and a quartet of methine at δ 2.27 in the ¹H-¹H COSY spectrum strongly supported the structure of the compound. The C=O carbon was confirmed by signal at 177.63 typical of a ketonic carbon. Thus, based on spectroscopic evidence, Compound 3 was identified as friedelin (Figure 3), and the spectral data is in agreement with the literature (Grasiely et al. 2012; Majidul et al. 2015).
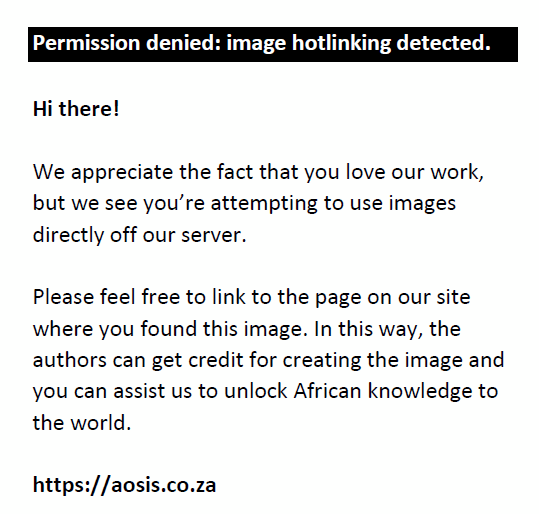 |
FIGURE 3: Friedelin isolated from L. capassa. |
|
¹H (CDCl3, 300 MHz, ppm): δH 1.61 (H-1, 2H,), 2.27 (Hb-2), 2.31 (Ha-2), 2.25 (H-4, 1H, m), 1.24 (H-6,), 0.84 (H-23, 3H), 0.80 (H-24, 3H), 0.84 (H-25, 3H), 1.00 (H-26, 3H), 1.02 (H-27, 3H), 1.03 (H-28, 3H), 1.20 (H-29, 3H).
13C NMR (CDCl3, 75 MHz, ppm): δC 22.65 (C-1), 33.65 (C-2), 177.63 (C-3), 56.00 (C-4), 39.50 (C-5), 36.33 (C-6), 14.16 (C-7), 55.50 (C-8), 39.5 (C-9), 59.52 (C-10), 33.65 (C-11), 30.8 (C-12), 35.64 (C-13), 39.52 (C-14), 31.95 (C-15), 39.51 (C-16), 29.39 (C-17), 43.1 (C-18), 35.9 (C-19), 29.27 (C-20), 33.65 (C- 21), 39.51 (C-22), 7.1 (C-23), 14.16 (C-24), 22.73 (C-25), 22.65 (C-26), 22.72 (C-27), 24.73 (C-28), 29.73 (C-29), 29.47 (C-30).
The chemical structure of Friedelin isolated from L. capassa is presented in Figure 3. Friedelin was previously isolated from Syzygium jambos (Majidul et al. 2015) and Maytenus robusta (Grasiely et al. 2012). Friedelin is used for the treatment of ulcerative colitis (Shi et al. 2021).
Compound 4
1H NMR: 5.47 (H-2), 3.61 (1H, H-3ax), 2.27 (H-3eq), 7.39 (1H, H-5), 6. (1H,H-6), 6.53 (2H, m, H-2’,6’), 6.41 (1H, H-5’), 6.08 (H-3”), 6.89 (1H, H-4”), 1.82, 1.81 (2 X 3H, 2 x s, 2”-Me2), 3.80, 3.83 (2 X 3H, 2 x s, 3’-OMe, 4’-OMe).
13C NMR: 79.49 (C-2), 39.37 (C-3), 157.25 (C-4), 132.25 (C-5), 125.91 (C-6), 156.84 (C-7), 103.66 (C-8), 152.21 (C-9), 112.51 (C-10), 135.59 (C-1’), 106.90 (C-2’), 149.46, 150.73 (C-3’, C-4’), 112.51 (C-5’), 120.51 (C-6’), 77.36 (C-2”), 125.91 (C-3”), 109.65 (C-4”), 31.94 (C-5’’), 125.91 (C-6’’) 28.83, 27.99 (2”-OMe), 55.61 (3’-OMe, 4’-OMe).
The compound 6-(γ,γ-Dimethylallyl)-3’,4’-dimethoxy-6”,6”-dimethylpyrano-[2”,3”:7,8]-flavanone in Figure 4 isolated from L. capassa is a new compound. Based on the literature, there is no information on 6-(γ,γ-Dimethylallyl)-3’,4’-dimethoxy-6”,6”-dimethylpyrano-[2”,3”:7,8]-flavanone.
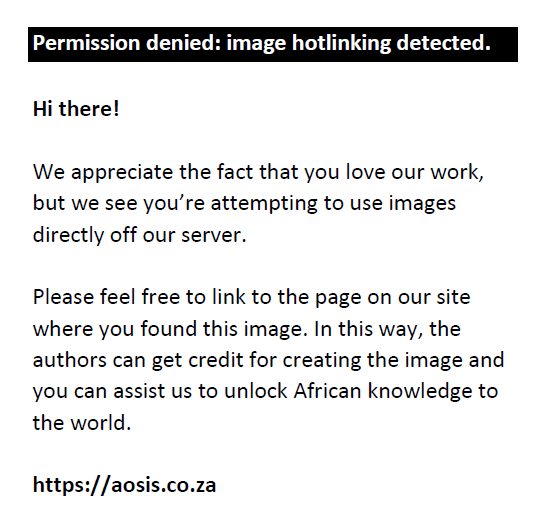 |
FIGURE 4: 6-(γ,γ-Dimethylallyl)-3’,4’-dimethoxy-6”,6”-dimethylpyrano-[2”,3”:7,8]-flavanone isolated from L. capassa. |
|
Antioxidant activity
Qualitative antioxidant activity
Strong antioxidant activity was visible in compounds Friedelin and 6-(γ,γ-Dimethylallyl)-3’,4’-dimethoxy-6”,6”-dimethylpyrano-[2”,3”:7,8]-flavanone when sprayed with 0.2% of DPPH in methanol; the compounds were visible in all the three-eluent systems BEA, CEF and EMW. No activity was observed in compound Lupeol, C2, and C5 compounds. Therefore, compounds Friedelin and 6-(γ,γ-Dimethylallyl)-3’,4’-dimethoxy-6”,6”-dimethylpyrano-[2”,3”:7,8]-flavanone were further analysed using the quantitative assay. It was not necessary to quantify compounds lupeol, Compound 2, and Compound 5 since no activity was observed on the TLC plate.
Quantitative antioxidant activity
There was a significant positive correlation between quantitative and qualitative assay of compounds Friedelin and 6-(γ,γ-Dimethylallyl)-3’,4’-dimethoxy-6”,6”-dimethylpyrano-[2”,3”:7,8]-flavanone. The isolated compounds were active against the DPPH by showing a high percentage of inhibition as presented in Figure 5. Compound 4 had percentage inhibition of 67% and 77% in 120 μg/mL and 250 μg/mL concentrations, respectively. Compound Friedelin also had a good inhibition percentage of 53% in 120 μg/mL and 54% in 250 μg/mL. However, it was low compared to that of compound 6-(γ,γ-Dimethylallyl)-3’,4’-dimethoxy-6”,6”-dimethylpyrano-[2”,3”:7,8]-flavanone. This shows that 6-(γ,γ-Dimethylallyl)-3’,4’-dimethoxy-6”,6”-dimethylpyrano-[2”,3”:7,8]-flavanone is highly active than the compound Friedelin in the quantitative assay.
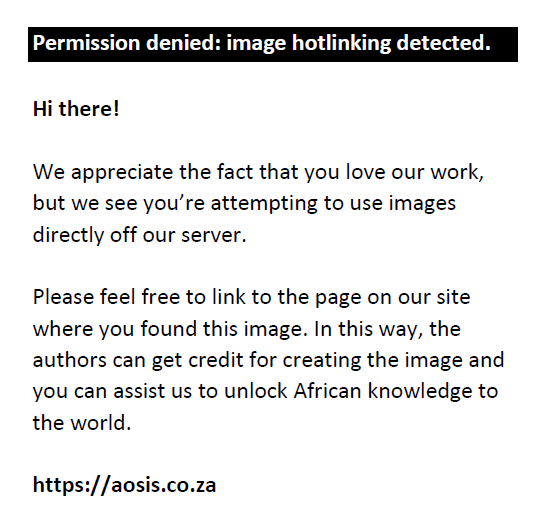 |
FIGURE 5: Percentage of antioxidant activity of isolated compounds and ascorbic acid. |
|
Cytotoxicity activity
The toxic effects of the acetone crude extract and isolated compounds were investigated using the MTT assay against the Vero monkey kidney cells. The acetone leaf extract was less toxic with LC50 of 0.23 mg/mL. Previously, it was reported that ethyl acetate bark and root extracts of L. capassa were toxic against brine shrimp LC50 of 17.86 μg/mL (Kilonzo et al. 2016). Compound 1 (Lupeol) and Compound 4 (6-(γ,γ-Dimethylallyl)-3’,4’-dimethoxy-6”,6”-dimethylpyrano-[2”,3”:7,8]-flavanone) were not toxic with LC50 ˃ 0.2 mg/mL against the Vero monkey cells. Elisha et al. (2017) reported that plant extracts with LC50 ˃ 0.2 mg/mL are relatively non-toxic.
Conclusion
The isolated compounds from the leaf extract of L. capassa were active against C. albicans, C. neoformans, and A. fumigatus. Interestingly, the antifungal activity of the isolated compounds was lower than that of the crude extracts indicating a possible synergistic effect between different compounds. Furthermore, some isolated compounds revealed good antioxidant activity in both scavenging assays, which indicates that isolated compounds of L. capassa can scavenge the free radicals causing fungal infections in humans. The study indicated that isolated compounds from L. capassa can be used as a source of therapeutic agents. The isolated compounds and crude extract of L. capassa were not toxic against the Vero monkey kidney cells, which ascertains the use of the plant extracts as medicine to treat fungal infections by traditional health practitioners. Nuclear Magnetic Resonance and Mass spectrometry were used for the identification of six isolated antifungal compounds from L. capassa leaves. However, only three compounds were successfully identified as Lupeol (Compound 1), Friedelin (Compound 3), and 6-(γ,γ-Dimethylallyl)-3’,4’-dimethoxy-6”,6”-dimethylpyrano-[2”,3”:7,8]-flavanone (Compound 4). Furthermore, 6-(γ,γ-Dimethylallyl)-3’,4’-dimethoxy-6”,6”-dimethylpyrano-[2”,3”:7,8]-flavanone was reported for the first time in this study.
Acknowledgements
The authors would like to acknowledge National Research Foundation (NRF) for financial support. They are grateful to the traditional health practitioners and local people who participated in this project.
Sections of this manuscript (12%) are published in a thesis submitted in partial fulfilment of the requirements for the degree of PhD and Masters in the University of Limpopo and University of Pretoria entitled ‘isolation, characterisation and cytotoxicity of antifungal compounds present in medicinal plants used against cryptococcus neoformans in vhembe district, limpopo province’ and ‘Ethnobotanical survey of medicinal plants with antifungal activities in Makhado Local Municipality, Limpopo Province, South Africa’ respectively with Supervisor: Prof. S. Mahlo, available here: http://ulspace.ul.ac.za/bitstream/handle/10386/4262/makhado_tc_2018.pdf?isAllowed=y&sequence=1 and http://ulspace.ul.ac.za/bitstream/handle/10386/4291/machaba_tc_2023.pdf?sequence=1&isAllowed=y.
Competing interests
The authors have declared that no competing interest exists.
Authors’ contributions
T.C.M. was involved in conceptualisation, methodology, formal analysis, investigation, writing – original draft, visualisation, data curation. S.M. contributed to conceptualisation, methodology, formal analysis, investigation, writing – original draft, visualisation, project administration, software, validation, data curation, resources, writing – review & editing, supervision, funding acquisition. J.E. was involved in conceptualisation, methodology, formal analysis, investigation, validation, data curation, writing – review & editing, supervision. W.N contributed to structure elucidation of the isolated compounds. L..M was responsible for cytotoxicity assay of the plant extracts and isolated compounds.
Funding information
The authors received no financial support for the research, authorship, and/or publication of this article.
Data availability
The data used to support the findings of this study is available from the corresponding author, S.M., upon reasonable request.
Disclaimer
The views and opinions expressed in this article are those of the authors and do not necessarily reflect the official policy or position of any affiliated agency of the authors, and the publisher.
References
Aberkane, A., Cuenca-Estreila, M., Gomez-Lopez, A., Petrikkou, E., Mellado, E., Monzon, A. et al., 2002, ‘Comparative evaluation of two different methods of inoculum preparation for antifungal susceptibility testing of filamentous fungi’, Journal of Antimicrobial Chemotherapy 50(5), 719–722. https://doi.org/10.1093/jac/dkf187
Adaramola, B. & Onigbinde, A., 2017, ‘Influence of extraction technique on the mineral content and antioxidant capacity of edible oil extracted from ginger rhizome’, Chemistry International 3(1), 1–7.
Ain, P.S. & Bari, S.B., 2010, ‘Isolation of Lupeol, Stigmasterol and Campesterol from petroleum ether extract of woody stem-bark of Wightia tinctoria’, Asian Journal of Plant Sciences 9(3), 163–167. https://doi.org/10.3923/ajps.2010.163.167
Ali, J.S., Riaz, N., Manna, A., Latif, M. & Zia, M., 2022, ‘Antioxidant, antimicrobial, enzyme inhibition and cytotoxicity guided investigation of Sideroxylon mascatense (A.D.C) T.D. Penn leave extracts’, Natural Product Research 36(16), 4227–4230. https://doi.org/10.1080/14786419.2021.1973461
Aumeeruddy-Elalfi, Z., Gurib-Fakim, A. & Mahomoodally, M.F., 2016, ‘Chemical composition, antimicrobial and antibiotic potentiating activity of essential oils from 10 tropical medicinal plants from Mauritius’, Journal of Herbs and Medicine 6(2), 88–95. https://doi.org/10.1016/j.hermed.2016.02.002
Begue, W.J. & Kline, R.M., 1972, ‘The use of tetrazolium salts in bioautographic procedures’, Journal of Chromatography 64(1), 182–184. https://doi.org/10.1016/S0021-9673(00)92965-0
Elisha, L.I., Jambalang, A.R., Botha, F.S., Buys, E.M., McGaw, L.J. & Eloff, J.N., 2017, ‘Potency and selectivity indices of acetone leaf extracts of nine selected South African trees against six opportunistic Enterobacteriaceae isolates from commercial chicken eggs’, BMC Complementary and Alternative Medicine 17, 90. https://doi.org/10.1186/s12906-017-1597-3
Fotie, J., Bohle, D.S., Leimanis, M.L., Georges, E., Rukungu, C. & Nkengfack, A.E., 2006, ‘Lupeol, long-chain fatty esters with antimalarial activity from Holarrhena floribunda’, Journal of Natural Products 69(1), 62–67. https://doi.org/10.1021/np050315y
Grasiely, F.S., Lucienir, P.D., Antonio, F.C.A., Gracia, D.F., Sidney, A.V., Roqueline, R.S. et al., 2012, ‘New triterpenes from Maytenus robusta: Structural elucidation based on NMR experimental data and theoretical calculations’, Molecules 17(11), 13439–13456. https://doi.org/10.3390/molecules171113439
Jain, P.S. & Bari, S.B., 2010, ‘Isolation of Lupeol, Stigmasterol and Campesterol from petroleum ether extract of woody stem-bark of Wightia tinctoria’, Asian Journal Plant Science 9(3), 163–167. https://doi.org/10.3923/ajps.2010.163.167
Kilonzo, M., Chacha, M. & Ndakidemi, P.A., 2017, ‘A review on antimicrobial efficacy, toxicity and phytochemicals of selected medicinal plants used in Africa against pathogenic microorganisms’, International Journal of Biosciences 10(3), 232–250. https://doi.org/10.12692/ijb/10.3.232-250
Kilonzo, M., Ndakidemi, P.A. & Chacha, M., 2016, ‘In vitro antifungal and cytotoxicity activities of selected Tanzanian medicinal plants’, Tropical Journal of Pharmaceutical Research 15(10), 2121. https://doi.org/10.4314/tjpr.v15i10.10
Kim, J., 2016, ‘Human fungal pathogens: Why should we learn?’, Journal of Microbiology 54(3), 145–148. https://doi.org/10.1007/s12275-016-0647-8
Kokwaro, J.O., 1993, Medicinal plant of East Africa, 2nd edn., p. 176, Kenya literature bureau, Nairobi.
Kotze, M. & Eloff, J.N., 2002, ‘Extraction of antibacterial compounds from Combretum microphyllum (Combretaceae)’, South African Journal Botany 68(1), 62–67. https://doi.org/10.1016/S0254-6299(16)30456-2
Lutta, K.P., Bii, C., Akenga, A.T. & Cornelius, W.W., 2008, ‘Antimicrobial marine natural products from the sponge Axinella infundibuliformis’, Records of Natural Products 2(4), 116–127.
Machaba, T.C., 2018, ‘Ethnobotanical survey of medicinal plants with antifungal activities in Makhado Local Municipality, Limpopo province, South Africa’, MSc dissertation, University of Limpopo.
Machaba, T.C., 2023, ‘Isolation, characterisation and cytotoxicity of antifungal compounds present in medicinal plants used against Cryptococcus neoformans in Vhembe District, Limpopo province’, PhD thesis, University of Limpopo.
Mahlo, S.M., McGaw, L.J. & Eloff, J.N., 2013, ‘Antifungal activity and cytotoxicity of isolated compounds from leaves of Breonadia salicina’, Journal of Ethnopharmacology 148(3), 909–913. https://doi.org/10.1016/j.jep.2013.05.041
Majidul, H.M., Marium, B., Moynul, H., Towheedur, R.M., Iftekhar, H., Mohammad, M.R. et al., 2015, ‘Investigation of the medicinal potentials of Syzygium jambos (L.) extract and characterization of the isolated compounds’, American Journal of Biological Science 3(2–1), 12–18. https://doi.org/10.11648/j.ajbio.s.2015030201.13
Margareth, B.C.G. & Miranda, J.S., 2009, ‘Biological activity of Lupeol’, International Journal of Biomedical Science 3(1), 46–66. https://doi.org/10.1080/09674845.2009.11730264
McGaw, L.J., Van Der Merwe, D. & Eloff, J.N., 2007, ‘In vitro anthelmintic, antibacterial and cytotoxic effects of extracts from plants used in South African ethnoveterinary medicine’, Veterinary Journal 173(2), 366–372. https://doi.org/10.1016/j.tvjl.2005.09.004
Masoko, P., Picard, J. & Eloff, J.N., 2005, ‘Antifungal activities of six South African terminalia species (Combretaceae)’, Journal of Ethnopharmacology 99(2), 301–308. https://doi.org/10.1016/j.jep.2005.01.061
Moshi, M.J., Kagashe, G.A.B. & Mbwambo, Z.H., 2005, ‘Plants used to treat epilepsy by Tanzanian traditional healers’, Journal of Ethnopharmacology 97(2), 327–336. https://doi.org/10.1016/j.jep.2004.11.015
Mosmann, T., 1983, ‘Rapid colorimetric assay for cellular growth and survival: Application to proliferation and cytotoxicity assays’, Journal of Immunology Methods 65(1–2), 55–63. https://doi.org/10.1016/0022-1759(83)90303-4
Samie, S., Trollopea, K.M., Jouberta, L.M., Makunga, N.P. & Volschenk, H., 2019, ‘The antifungal and Cryptococcus neoformans virulence attenuating activity of Pelargonium sidoides extracts’, Journal of Ethnopharmacology 235, 122–132. https://doi.org/10.1016/j.jep.2019.02.008
Sanguinetti, M., Posteraro, B. & Lass-Florl, C., 2015, ‘Antifungal drug resistance among Candida species: Mechanisms and clinical impact’, Mycoses 58(S2), 2–13. https://doi.org/10.1111/myc.12330
Saxena, H.O., Mohan, B., Kakkar, A. & Pawar, G., 2017, ‘Chemotypic variation of Lupeol in roots of Hemidesmus indicus (L.) R. Br from different agroclimatic regions of Medhya Pradesh state of India’, Current Traditional Medicine 3(1), 29–37. https://doi.org/10.2174/2215083802666161104154139
Saxena, H.M., Parikar, S., Pawar, G. & Rao, G.R., 2023, ‘Simultaneous densitometric determination of β-sitosterol and Lupeol through validated HPTLC method in different plant parts of Uraria picta (Jacq.) Desv.ex DC.-A dashmool species’, Acta Chromatographica 35(1), 99–105. https://doi.org/10.1556/1326.2022.01018
Schmiedel, Y. & Zimmerli, S., 2016, ‘Common invasive fungal disease: An overview of invasive candidiasis, aspergillosis, cryptococcosis, and pneumocystis pneumonia’, Current Opinion 146(708), 14281. https://doi.org/10.4414/smw.2016.14281
Shi, B., Liu, S., Huang, A., Zhou, M., Sun, B., Cao, H. et al., 2021, ‘Reaving the mechanism of Friedelin in the treatment of ulcerative colitis based on network pharmacology and experimental verification’, Evidence-Based Complementary and Alternative Medicine 2021, 4451779. https://doi.org/10.1155/2021/4451779
Stahl, E., 1969, Apparatus and general techniques in TLC. Thin-Layer Chromatography, pp. 52–86, Springer-Verlag, Berlin.
Vallabhaneni, S., Haselow, D., Lloyd, S., Lockhart, S., Moulton-Meissner, H., Lester, L., Wheeler, G. et al., 2016, ‘Cluster of Cryptococcus neoformans infections in intensive care unit, Arkansas, USA’, Emerging Infectious Diseases 21(10), 1719–1724. https://doi.org/10.3201/eid2110.150249
Van Wyk, A.K. & Van Wyk, P., 1997, Field guide to trees of Southern Africa, Struik, Cape Town.
Vendruscolo, I., Venturell, S.R.T., Bressiani, P.A., Marco, I.G., Novello, C.R., Almeida, I.V. et al., 2022, ‘Cytotoxicity of extracts and compounds isolated from Croton echiodes in animal tumour cell HTC’, Brazilian Journal of Biology 82, 264356. https://doi.org/10.1590/1519-6984.264356
Wal, P., Wal, A., Sharma, G. & Rai, A.K., 2011, ‘Biological activities of Lupeol’, Systematic Review Pharmarcy 2(2), 96–103. https://doi.org/10.4103/0975-8453.86298
Zhang, A., Sun, H., Yan, G. & Wang, X., 2017, ‘Recent developments and emerging trends of mass spectrometry for herbal ingredients analysis’, TrAC Trends in Analytical Chemistry 94, 70–76. https://doi.org/10.1016/j.trac.2017.07.007
Zhang, M., Yan, H., Lu, M., Wang, D. & Sun, S., 2020, ‘Antifungal activity of ribavirin used alone or in combination with fluconazole against Candida albicans is mediated by reduced virulence’, International Journal Antimicrobial Agents 55(1), 105804. https://doi.org/10.1016/j.ijantimicag.2019.09.008
|